Blood typing

Background
Blood typing is a test that determines a person’s blood type by analyzing donor-recipient compatibility with blood group antigens and antibodies. If you are in need of a blood transfusion or if you plan to be a donor is a crucial testing. It allows for the identification and confirmation of Rh- women, improving the design of preconceptional and prenatal care.
Although ABO and Rh are the major groups, a total of 43 blood systems containing 345 antigens for human red blood cells were identified at the time of this review (1). This results in other tests being necessary in specific cases, such as a screening test for antibodies against RBCs from rare systems, accompanied by a crossmatch to determine which blood components can be safely administered.
History
The ABO system was first discovered in 1900 by Karl Landsteiner by cross-matching red blood cells with sera from different individuals. It was subsequently classified into five groups as per their glycoprotein antigens (A, B, AB, A1, and H) expressed on the surface of red blood cells (Figure 1). (1)
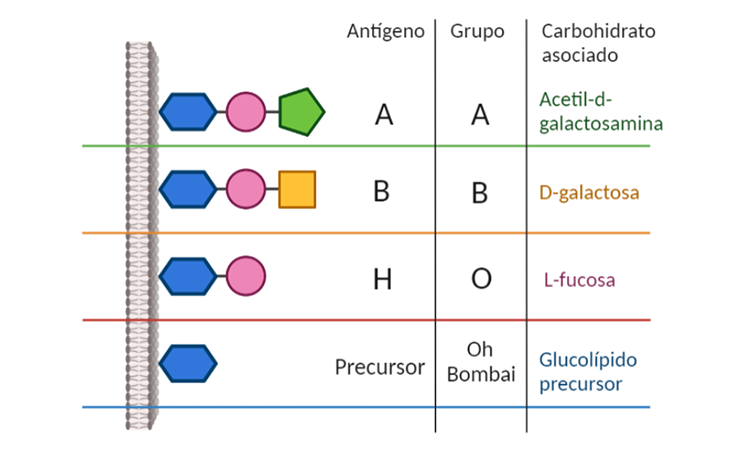
Figure 1. The ABO System (Spanish).
Genetics of the ABO System
The H gene, located on chromosome 19, codes for the production of the H transferase enzyme, which binds an L-fucose molecule to the terminal galactose (Gal) of a common precursor bound to erythrocyte membrane lipids or proteins, giving rise to the H antigen, which is the initial step in the formation of ABO blood group antigens.
For H antigen production, there must be at least one functional copy of the H gene (H/H or H/h); if both copies of the gene are inactive (h/h), the Bombay phenotype is developed. This explains why the serum of patients with Bombay erythrocytes has antibodies against the H antigen, which react with A, B, AB and O cells. Therefore, people with the Bombay phenotype should be transfused only with erythrocytes of the Bombay phenotype. (2)
The ABO gene located in chromosome 9, has three alleles: A, B and O. Each allele varies according to nucleotide substitutions that determine the specificities of the enzymes for which they encode. (2)
The alleles coding for the ABO group are expressed in 10 possible combinations or genotypes (Table 1). Alleles A1 and A2 are codominant in relation to B and show complete dominance over the recessive allele O. Allele A1 is dominant over A2.
Genotype | Fenotype |
---|---|
A1A1 | A1 |
A1A2 | A1 |
A1O | A1 |
A2A2 | A2 |
A2O | A2 |
BB | B |
BO | B |
A1B | A1B |
A2B | A2B |
OO | O |
Table 1. Different combinations of the ABO gene with their corresponding phenotype.
Rhesus System (Rh)
The Rh system was discovered in the 1940s. It is one of the most polymorphic and antigenic blood group systems. It is also a major cause of hemolytic disease of the fetus and newborn, and second only to the ABO system in importance in transfusion.
The main antigen is D, and the term Rh positive and Rh negative refers to the presence or absence of the D antigen. Other common Rh antigens are C, c, E and e. Patients are not usually typed for these antigens unless they have developed atypical antibodies or must undergo long-term transfusions.
Two genes RHD and RHCE located on the short arm of chromosome 1, encode for Rh proteins. The first encodes the D antigen and the other encodes the CE antigens in various combinations (ce, cE, Ce or CE). The majority of D negative (Rh negative) phenotypes are the result of complete deletion of the RHD gene (3).
The D antigen is highly antigenic and prior to the routine use of Rh immunoglobulin, anti-D antibody was the only clinically significant antibody most frequently observed in pretransfusion testing.
Roughly 2% of individuals of European origin have changes in the RHD gene. These modifications encode structural changes in the RhD antigen, which usually cause weak RhD and partial RhD phenotypes.
The weak expression of D antigen is mainly found in people with a single RHD gene that has a mutation coding for an amino acid change. This causes some red blood cells with extremely low levels of D antigen to go undetected by routine typing methods.
RBCs with partial D antigen result from the inheritance of hybrid genes in which portions of RHD are replaced by the corresponding portions of RHCE. This results in the loss of some D epitopes. Although the red blood cells are typed as D positive, these patients generate anti-D antibodies following transfusion or pregnancy.
The C and c antigens are encoded by alternative forms of the RHCE gene and are codominant with each other, with equal expression in Cc genotypes. E and e antigens are co-dominant, and in all populations e is more frequent than E. (3)
General Principles of blood group identification tests
The presence of ABO/RhD antigens on the cell surface is associated with the absence of antibodies specific for that antigen. Table 2 shows the possible combinations of antibodies and antigens detected in the same patient for the ABO/RhD systems.
Group | Anti-A | Anti-B | Anti-AB | Anti-D |
---|---|---|---|---|
A+ | - | + | + | - |
A- | - | + | + | + |
B+ | + | - | + | - |
B- | + | - | + | + |
AB+ | - | - | - | - |
AB- | - | - | - | + |
O+ | + | + | + | - |
O- | + | + | + | + |
Table 2. Combinations of antibodies and antigens of the ABO/Rh System.
The principles of blood group identification are mostly based on antigen-antibody reactions. Various antibodies react with specific surface antigens causing some degree of agglutination. This result is interpreted as positive for the phenotype of the expressed surface antigen (e.g. A+).
On the other hand, crossmatching tests are based on the reaction of surface antigens present in the red blood cells of potential donors with antibodies present in the plasma of a recipient. If the crossmatch test is positive it expresses donor-recipient incompatibility. Systems other than ABO/Rh can cause a positive crossmatch, hence its clinical importance in avoiding post-transfusion reactions.
Blood group identification test methods
Plate agglutination test
The plate agglutination test is a simple and rapid method (5 to 10 minutes) to determine blood groups using small amounts of reagents. Despite its speed, this technique has several disadvantages: rare or weakly expressed antigens cannot be easily detected, while low titers of anti-A or anti-B antibodies often lead to false-negative results. It is therefore routinely used only in emergency cases.
Tube agglutination test
In comparison to the plate agglutination test, the tube agglutination test is more sensitive and reliable, therefore, it can be conveniently used for blood transfusions. To perform the test, the mixture of red blood cells and antibodies is centrifuged and the result is easily interpreted by visual examination. If the sediment of red cells in the test tube is not disturbed after centrifugation for a few minutes and gentle shaking, it is considered a complete agglutination (Figure 1).
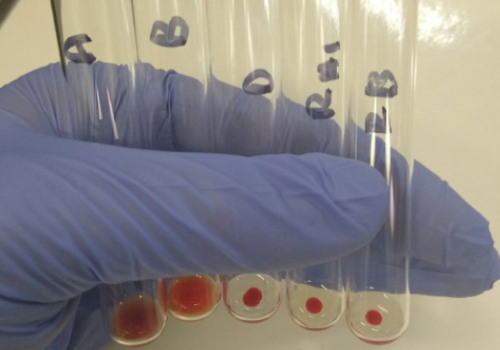
Figure 1. Tube agglutination test, O+ patient. Copyright - Wikimedia.
In case of obvious lack of agglutination, a small amount of RBC suspension is deposited on a glass slide and the degree of agglutination is observed under the microscope (Figure 2).
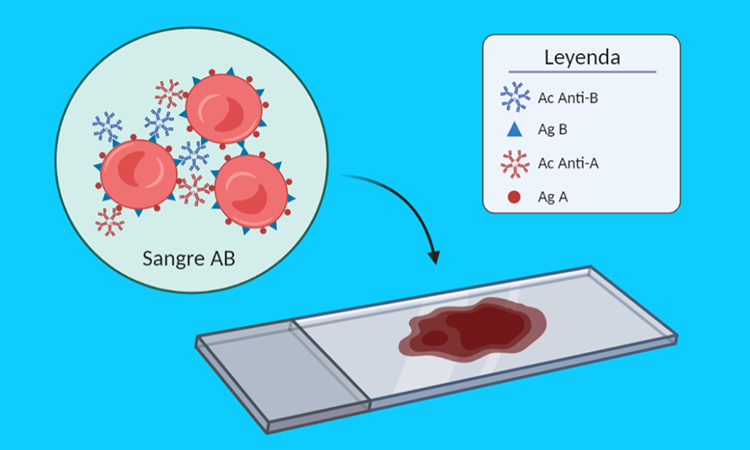
Figure 2. Schematic representation of erythrocyte agglutination in type AB blood sample. Created with Biorender.
Microplate agglutination test
Microplate technology uses automated platforms to detect serum antibodies and surface antigens on red blood cells. Reagents and red blood cells are centrifuged and incubated in microplates, and the ABO/RhD blood type is interpreted by an automated system. It has the advantages of speed, low reagent consumption and high throughput analysis.
The U-shaped microplate agglutination method (Figure 3) involves the addition of plasma and red blood cells, centrifugation, incubation with constant agitation, and final observation and interpretation. It is one of the most widely used as it can detect multiple blood groups from several patients at the same time. The appearance of red blood cell clots or fine sand in the U-shaped micropores indicates agglutination.
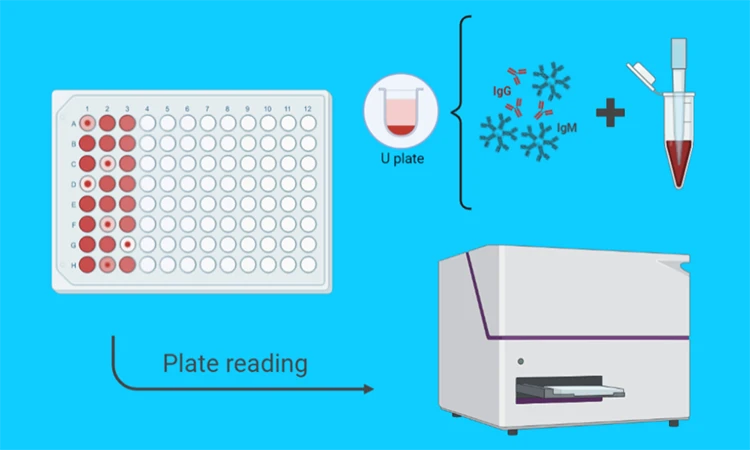
Figure 3. U-shaped microplate agglutination test. Created with Biorender.
Gel microcolumn agglutination test
Gel microcolumn agglutination technology was introduced by Lapierre et al. (4) in 1990. The automated version is the standard method for blood group typing in clinical laboratories and blood banks. The microcolumn is made of small microtubes containing gel matrix to trap agglutinates.
Blood serum or cells are mixed with anti-A, anti-B and anti-D reagents in microtubes under controlled incubation and centrifugation. Gel particles trap agglutinates, while unbound blood cells can pass through the column (Figure 4). (5)
The test time can be reduced by using glass particles instead of gel, as this achieves faster spin speeds. Some advantages of this method are that small amounts of reagents are used and it has high sensitivity, precision and reproducibility, which helps to reduce identification errors caused by human factors.
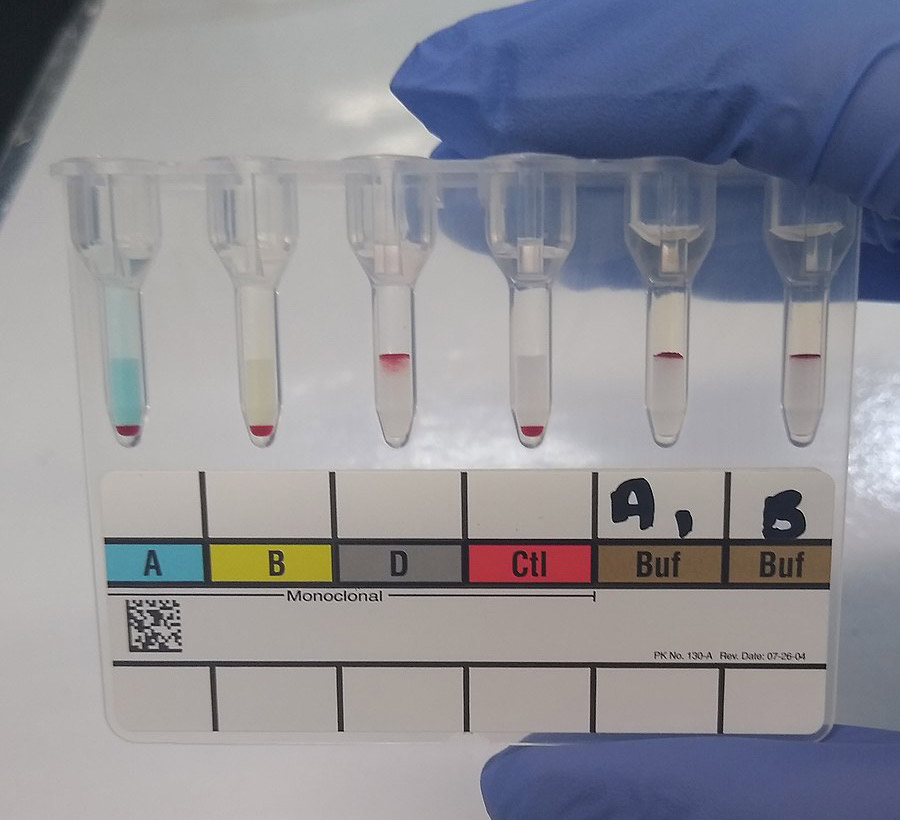
Figure 4. Gel microcolumn agglutination test, O+ patient. Copyright - Wikimedia.
Dual agglutination test on paper (ABO/Rh)
Paper-based diagnostic devices are becoming increasingly popular due to their ability to perform rapid, low-cost diagnostic tests in an out-of-laboratory setting and high patient convenience, like the family physician’s office.(6)
One of the most notorious techniques of this technology was developed by Noiphung et al. (7), who adapted the red blood cell agglutination approach using antibodies immobilized on a paper strip. For this purpose, researchers developed a paper-based assay that simultaneously performed direct and inverse determination of ABO and Rhesus groups.
The test design consisted of three gridded channels for direct clustering, two of which were also used for reverse clustering (Figure 5). The device was fabricated using chromatography paper and plasma separation membrane with combined printing and wax immersion methods.
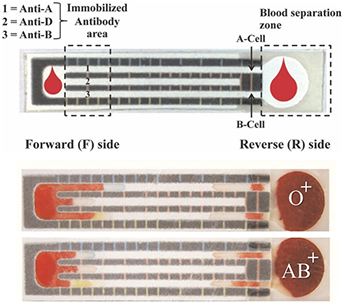
Figure 5. Paper agglutination. Copyright - Ebrahimi Fana, S et al. Biomed Microdevices. 2021.
In forward side pooling, the 1/2 diluted blood sample was applied to the front side of the device (chromatography paper) and flowed into the three channels pre-impregnated with anti-A, anti-B and anti-D antibodies.
In reverse side pooling, undiluted blood was applied to the reverse side of the device (plasma separation membrane). After separation, plasma flowed into the two channels impregnated with type A RBCs and type B RBCs.
This cost-effective and robust assay proved to be useful for environments with limited time and resources.
Dye paper agglutination test
This method developed by Zhang, H. et al. (8) utilizes linter paper as matrix and the dye bromocresol green. Depending on the presence or absence of agglutination, this dye undergoes a specific color change in the reaction zone.
The paper strip can accurately detect ABO/Rh blood groups in 30 seconds and determine 16 rare blood groups in 3 minutes. In addition, it has a fast error discrimination platform and a color correction algorithm. This method is the test of choice in emergency cases (Figure 6).
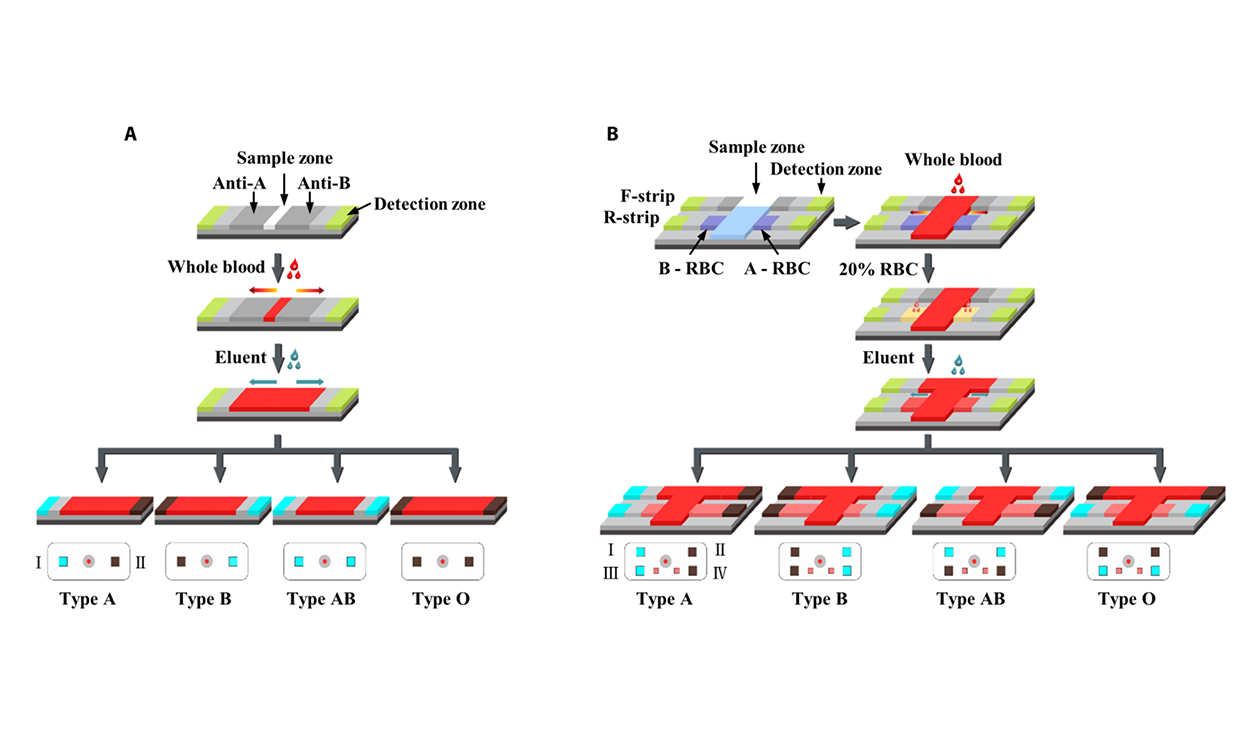
Figure 6. Dye paper agglutination test. Copyright - Zhang, H. et al. Science Translational Medicine. (8)
Microfluidic devices
These devices rely on digital microfluidization, which manipulates discrete droplets at the nanometer or microscopic scale in an open electrode array by increasing the electrical potential. (9)
The test consists of 2 plates, a lower plate containing a set of actuation electrodes used to manipulate drops containing test reagents or blood samples, and an upper plate that acts as an opposing electrode.
Hemagglutination assays are performed by dosing droplets of whole blood into the electrode array, where they are mixed with drops of agglutinating antibodies (blood typing), donor plasma (compatibility) or chemistry agents (hematocrit).
For blood typing, the anti-A, anti-B, anti-AB or anti-D antibodies are each mixed with separate droplets of whole blood (Figure 7).
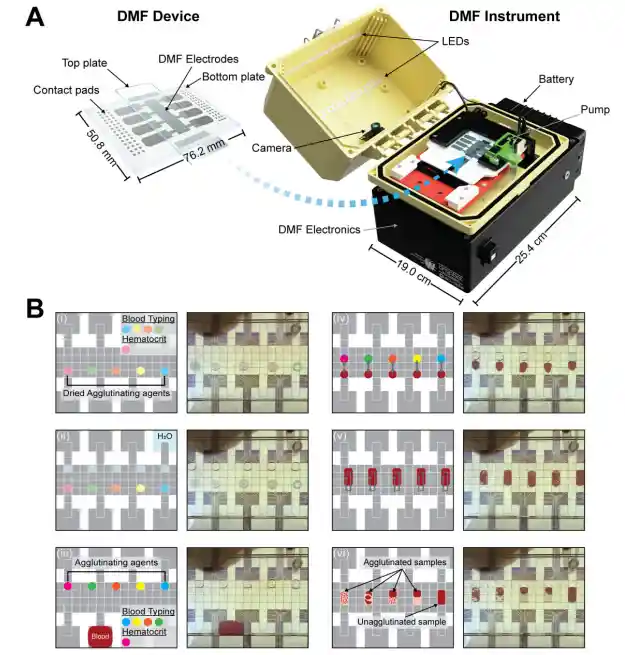
Figure 7. Agglutination in microfluidic devices. Copyright - Sklavounos AA et al. Clin Chem. (9)
The advantages of this technique are that samples can be collected, processed and quantified by an automated system, using simple portable instruments that can operate in remote and difficult environments with limited access to centralized laboratories. Another advantage is that donor compatibility can be detected by performing a paired test on the same device.
Waveguide-based sensors
A waveguide-based sensor can detect particles and molecules on the sensor chip using electric field potentiation with waveguide modulations.
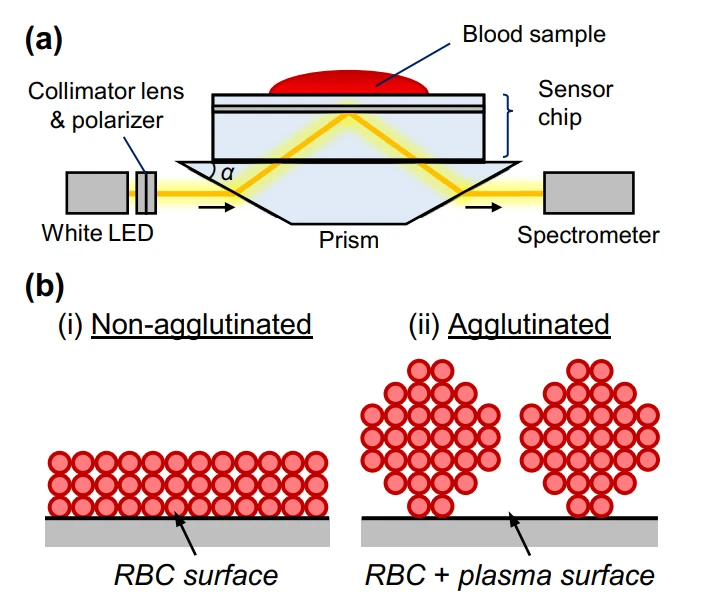
Figure 8. Working principle of waveguide-based sensors. Copyright - Ashiba, H. et al. Sensing and Bio-Sensing Research. (10)
This method of hemagglutination detection provides a simple form of blood typing based on optical sensors using a portable instrument. The test is as simple as the plate agglutination test, with potentially higher sensitivity.
Erythrocyte-magnetized technology (EMT)
It is an automated technology for the determination of ABO/Rh and antibody systems based on the magnetization of red blood cells with magnetic particles.
These particles are attached to the red blood cell surface by adsorption or by binding to glycoprotein A (GPA). Adsorptive binding employs positively charged iron particles, which bind to the negatively charged molecules present on the surface of the red blood cell. Binding to GPA is mediated by a specific anti-GPA antibody associated with an iron particle (Figure 9). (11)
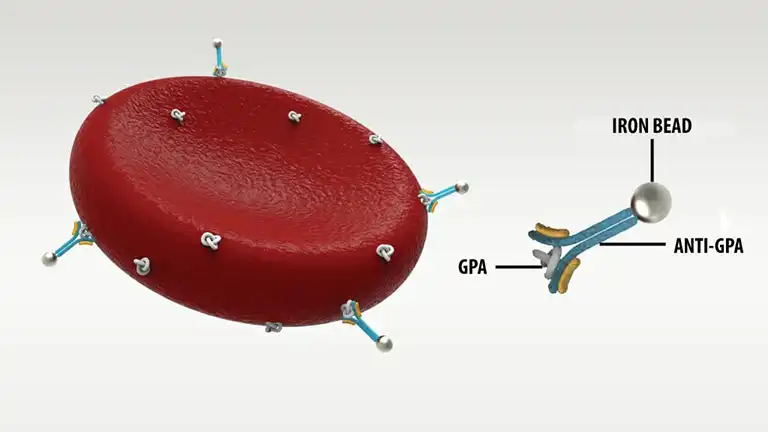
Figure 9. Erythrocyte-magnetized technology (GPA Binding). Copyright - DIAGAST E.M.® Technology
When exposed to a magnetic field in the microplate, the red blood cells migrate to the bottom of each well. Thereby the antigen-antibody reaction is enhanced (Figure 10). This effect eliminates the centrifugation step during the analytical process.
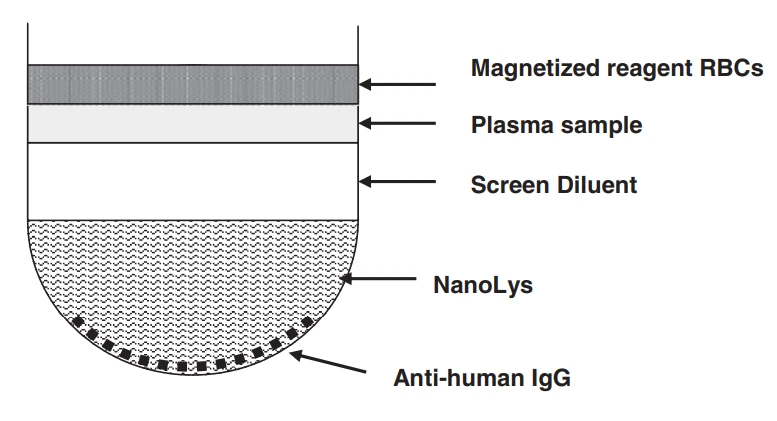
Figure 10. Erythrocyte-magnetized technology. Copyright - Bouix, O. et al. Transfusion. (12)
Plasmon resonance technology
Surface plasmon resonance (SPR) is a sensitive technique for tracking molecular interactions in real time. Specific IgM or IgG antibodies to antigens of the ABO system on the surface plasmon can generally be used to identify blood groups. The intensity of agglutination depends on the adhesion of red blood cells and their ability to dissociate (Figure 11).
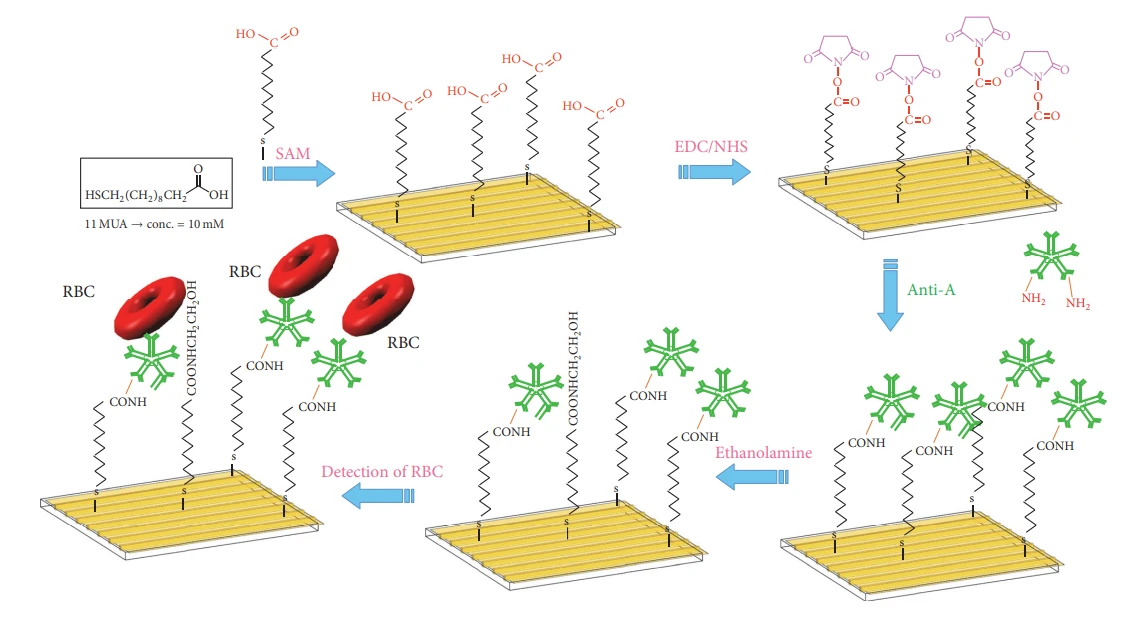
Figure 11. Immobilized erythrocytes by plasmon resonance technology. Copyright - Wanida Tangkawsakul et al. International Journal of Analytical Chemistry. (13)
SPR can determine the ABO/Rh blood group in 12 minutes. The sensor chip can quantify the presence of blood group surface antigens, which could support the detection of weaker variants. However, SPR requires expensive equipment and consumables and may not be practical for routine blood group testing.(13)
Conclusions
New technologies for the analysis of blood groups are continuously emerging. Some of them can accurately detect minor variants. However, most new tests are time-consuming and costly.
Serological methods are still routinely used for ABO and Rh typing. These methods and the ones to come, are ensuring safe blood transfusions in all clinical settings.
References
- Li, H., & Guo, K. (2022). Blood Group Testing. Frontiers in Medicine, 9. https://doi.org/10.3389/fmed.2022.827619
- Arbeláez García, CA. Sistema de grupo sanguíneo ABO. Medicina & Laboratorio, 15(7-8), 2009.
- Vege, S., & Westhoff, C. M. (2019). Rh and RhAG Blood Group Systems. Transfusion Medicine and Hemostasis, 149–155. https://doi.org/10.1016/b978-0-12-813726-0.00026-x
- Lapierre Y, Rigal D, Adam J, Josef D, Meyer F, Greber S, et al. The gel test: a new way to detect red cell antigen-antibody reactions. Transfusion. (1990) 30:109–13. https://doi.org/10.1046/j.1537-2995.1990.30290162894.x
- Mujahid, A., & Dickert, F. (2015). Blood Group Typing: From Classical Strategies to the Application of Synthetic Antibodies Generated by Molecular Imprinting. Sensors, 16(1), 51. https://doi.org/10.3390/s16010051
- Hua Li and Andrew J. Steckl Analytical Chemistry 2019 91 (1), 352-371. https://doi.org/10.1021/acs.analchem.8b03636
- Noiphung et al., A novel paper-based assay for the simultaneous determination of Rh typing and forward and reverse ABO blood groups. Biosens. Bioelectron. 67, 485–489 (2015). https://doi.org/10.1016/j.bios.2014.09.011
- Zhang, H., Qiu, X., Zou, Y., Ye, Y., Qi, C., Zou, L., … Luo, Y. (2017). A dye-assisted paper-based point-of-care assay for fast and reliable blood grouping. Science Translational Medicine, 9(381), eaaf9209. https://doi.org/10.1126/scitranslmed.aaf9209
- Sklavounos AA, Lamanna J, Modi D, Gupta S, Mariakakis A, Callum J, et al. Digital microfluidic hemagglutination assays for blood typing, donor compatibility testing, and hematocrit analysis. Clin Chem. (2021) 67:1699–708. https://doi.org/10.1093/clinchem/hvab180
- Ashiba, H., Fujimaki, M., Awazu, K., Fu, M., Ohki, Y., Tanaka, T., & Makishima, M. (2015). Hemagglutination detection for blood typing based on waveguide-mode sensors. Sensing and Bio-Sensing Research, 3, 59–64. https://doi.org/10.1016/j.sbsr.2014.12.003
- Patel TR, Shah SD, Bhatnagar N, Shah MC, Tripathi S, Soni S, et al. The evaluation of M‑TRAP technology in detection of ABO groups and its subgroups and its efficacy as a point‑of‑care test for blood grouping. Glob J Transfus Med 2021;6:150-5.
- Bouix, O., Ferrera, V., Delamaire, M., Redersdorff, J. C., & Roubinet, F. (2008). Erythrocyte-magnetized technology: an original and innovative method for blood group serology. Transfusion, 48(9), 1878–1885. https://doi.org/10.1111/j.1537-2995.2008.01790.x
- Wanida Tangkawsakul, Toemsak Srikhirin, Kazunari Shinbo, Keizo Kato, Futao Kaneko, Akira Baba, “Application of Long-Range Surface Plasmon Resonance for ABO Blood Typing”, International Journal of Analytical Chemistry, vol. 2016, Article ID 1432781, 8 pages, 2016. https://doi.org/10.1155/2016/1432781